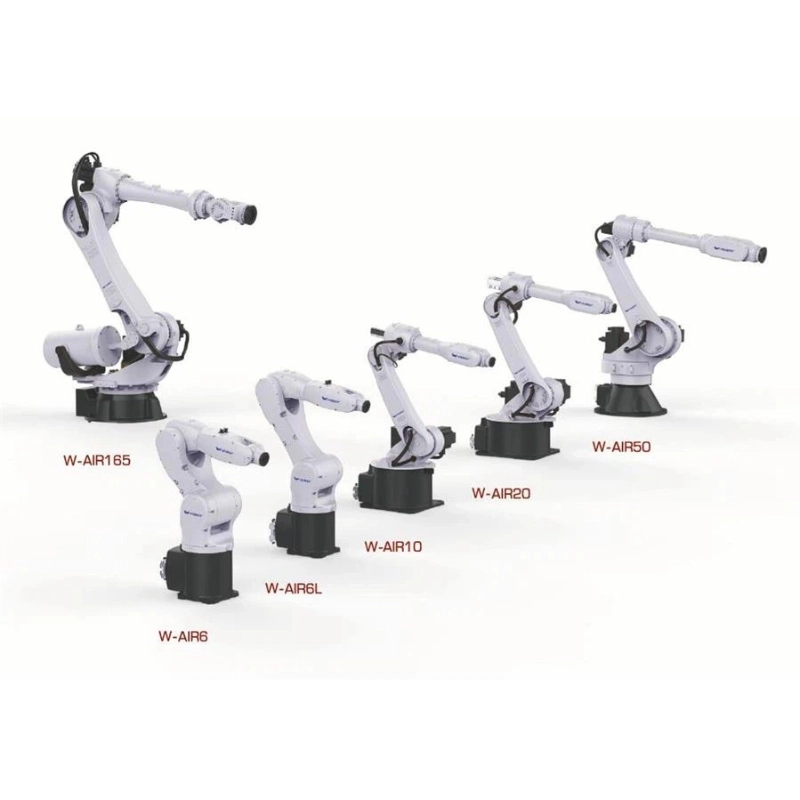
Six Joint Robot
2023-05-12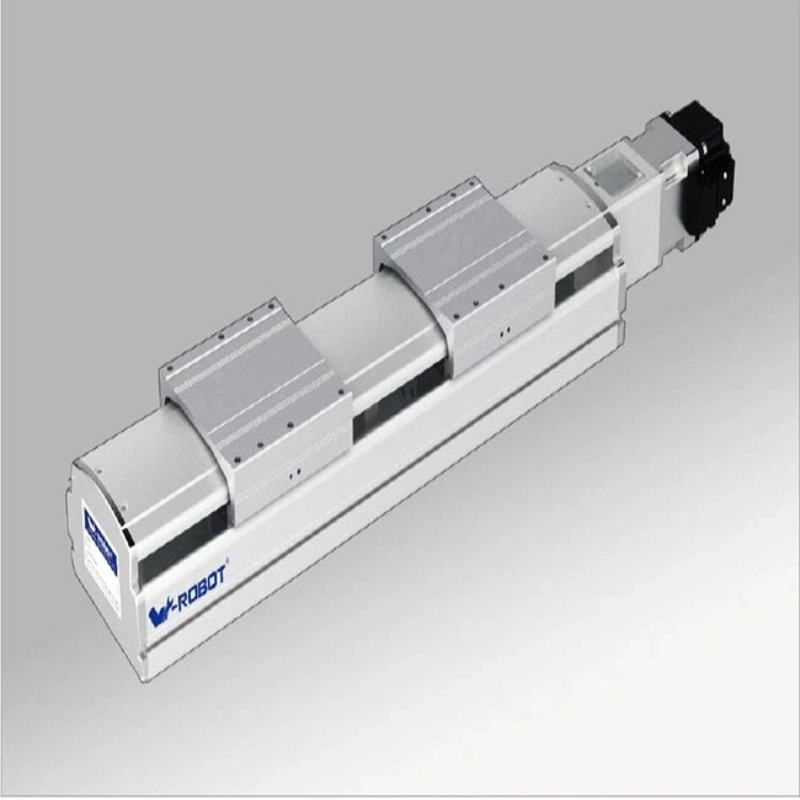
Clamp Type Single Robot
2023-05-12Scara Electric Robot
The six-joint industrial robot has a high degree of freedom, six joints, and a long travel, which is suitable for almost any trajectory or angle of work.
1. Product Introduction
The SCARA acronym stands for Selective Compliance Assembly Robot Arm or Selective Compliance Articulated Robot Arm.
SCARA Robots are a popular option for small robotic assembly applications.
SCARA is an acronym for Selective Compliance Articulated Robot Arm, meaning it is compliant in the X-Y axis, and rigid in the Z-axis.
SCARA configuration is unique and designed to handle a variety of material handling operations.
By virtue of the SCARA’s parallel-axis joint layout, the arm is slightly compliant in the X-Y direction but rigid in the ‘Z’ direction, hence the term: Selective Compliant. This is advantageous for many types of assembly operations, i.e., inserting a round pin in a round hole without binding.
The second attribute of the SCARA is the jointed two-link arm layout similar to our human arms, hence the often-used term, Articulated. This feature allows the arm to extend into confined areas and then retract or “fold up” out of the way. This is advantageous for transferring parts from one cell to another or for loading/ unloading process stations that are enclosed.
SCARAs are generally faster than comparable Cartesian robot systems. Their single pedestal mount requires a small footprint and provides an easy, unhindered form of mounting. On the other hand, SCARAs can be more expensive than comparable Cartesian systems and the controlling software requires inverse kinematics for linear interpolated moves. This software typically comes with the SCARA though and is usually transparent to the end-user.
Most SCARA robots are based on serial architectures, which means that the first motor should carry all other motors. There also exists a so-called double-arm SCARA robot architecture, in which two of the motors are fixed at the base.
2. Product Parameter (specification)
3. Product feature and application:
What Can You Do With a SCARA Robot?
Do you need a SCARA or another type of robot? With a new SCARA added to our Robot Library, here are the benefits of this classic robot.
What are the benefits of them over the more common 6-axis robots?
As the performance of 6-axis robots continues to improve year after year, you might be wondering: Do I need a SCARA?
Let’s take a look at this classic robot and find out what you can do with it.
SCARA is an acronym, but the meaning of the first letter A has changed slightly over the years.
The two definitions are:
Selective Compliance Assembly Robot Arm — This was the original definition and is still probably the most common.
Selective Compliance Articulated Robot Arm — This definition came into use later as SCARAs have been applied to other tasks which do not involve assembly.
These days, SCARAs are used for a whole host of different industrial tasks in a wide range of industries.
What do those other terms mean?
The final two letters (RA for “Robot Arm”) are self-explanatory, but what about Selective Compliance?
Selective Compliance
In robotics, compliance means that a robot has flexibility in one or more of its joints (or sometimes in its mechanical structure). If you push a compliant robot, it will give way under your touch. It won’t push back or hold steady.
SCARAs are compliant in the X-Y axis but rigid in the Z-axis. This gives them some flexibility which is particularly useful for assembly applications which require compliance— e.g. inserting a peg in a hole.
How a SCARA Compares to Other Robots
These days, there are so many different robots with different levels of performance. It is difficult to say that SCARAs are “better” than other types in any particular aspect. There is often more variability between individual robot models than between robot types.
Even so, here are six categories where SCARAs tend to be different from other robot types.
Rigidity
Due to their selective compliance, SCARAs are less rigid than Cartesian or gantry robots. However, they are more rigid than both 6-axis robots and Delta robots due to their rigid Z-axis.
This makes SCARAs a kind of “halfway house” between Cartesian and 6-axis robots.
Speed
SCARAs are very well suited to high-speed assembly applications. They are generally faster than 6-axis robots. As a result, it is much more likely to see them being used for pick-and-place. However, they are not as fast as Delta robots which are the top choice for high-speed pick-and-place.
Axes
One of the clearest distinctions between SCARAs and 6-axis robots is that they have fewer Degrees-of-Freedom. Like most Delta robots, they have only 4 axes.
Payload
The payload of SCARAs is generally quite low. The models in our Robot Library can lift between 0.5-20 kg. This is much less than 6-axis robots which can lift between 1-1700 kg, but it is more than Delta robots which can lift between 0.3-8 kg.
Repeatability
Assembly tasks require quite a high degree of precision, which means that SCARAs generally have better repeatability than other types of robot. The robots with the best repeatability in our Robot Library are all SCARAs and it’s not uncommon to see them with a repeatability of just 0.01 mm.
Cost
When SCARAs were first introduced in the 1980s, they represented the best price to performance ratio for high-speed tasks. This is still true today as they tend to be cheaper than the faster Delta robots.
Tasks That SCARA Robots Excels At
Robots are very adaptable and almost every type of robot can be used for a variety of tasks. SCARAs are no different but there are some tasks that they really excel at. Here are five of them:
A. Small Assembly
The task that the SCARA was designed to do. High-speed assembly tasks, such as those in the electronics industry, are very common for SCARA robots. Their selective compliance means that they can perform insertions more easily than other types of robot, without having to use complex programming.
B. Pick and Place
SCARAs are often the quickest, cheapest robot for high-speed pick and place. Their speed is only beaten by Delta robots, but they are usually easier to install because they do not require the robot to be mounted above the workspace.
C. Laser Engraving
SCARA robot drawing application
The high precision of SCARAs means that they are also very well suited to laser engraving and drawing tasks. Over the last few years, several end effectors have come onto the market which allow you to add laser engraving capabilities to any small robot.
D. 3D Printing
Another task which is becoming a rising star in the world of robotics is 3D printing. We’ve already seen a lot of applications where 6-axis robots are making it possible to print much bigger objects with 3D printing technology. SCARAs are well suited to this new application on the smaller end of the scale.
E. Soldering
SCARAs are very popular in the electronics industry because they can be used for many of the core manufacturing tasks. One such task is soldering. With a suitable end effector, they can provide very consistent quality of solder and improve efficiency when compared to soldering by hand.
4. Production detail:
5. Product qualification:
6. Deliver, shipping and serving:
7. FAQ:
The Difference between Cartesian, Six-Axis, and SCARA Robots
Originally, robots only automated manufacturing lines outputting high volumes. Now, robots execute tasks in smaller-scale applications, because implementing them is easier than ever. Here’s how Cartesian robots compare with the other two robot types.
The use of Cartesian and six-axis robots, as well as selective-compliance-articulated robot arms (SCARAs) is on the rise. They automate tasks to accelerate cycle times, increase throughput, and eliminate bottlenecks. Advanced controls make the robots user-friendlier than ever, with fewer backend-programming requirements. And in some cases, online tools let end users and OEMs quickly choose and configure robot features.
This packaging design uses a robot that picks boxes off a high-speed conveyor and places them on a pallet. The picker must extend a full meter to grab the boxes and move them down to the floor and onto a pallet, making for a cantilever situation. Therefore, a cost-effective Cartesian robot makes the most sense. Robots of all types are spreading to new and creative applications, but Cartesian robots in particular are proliferating thanks to linear servomotors, modules, standardized components, and operator-friendly controls that lower cost and boost performance.
Cartesian robots, sometimes called gantry robots, are mechatronic devices that use motors and linear actuators to position a tool. They make linear movements in three axes, X, Y, and Z. Physical scaffolding forms a framework that anchors and supports the axes and payload. Certain applications, such as machining tightly toleranced parts, require full support of the base axis, usually the X axis. In contrast, other applications, such as picking bottles off a conveyor, require less precision, so the framework only needs to support the base axis in compliance with the actuator’s manufacturer recommendations. Cartesian-robot movements stay within the framework’s confines, but the framework can be mounted horizontally or vertically, or even overhead in certain gantry configurations.
This file type includes high resolution graphics and schematics when applicable.
In contrast, SCARAs and six-axis robots typically mount on a pedestal. SCARAs move in the X, Y, and Z planes like Cartesians, but incorporate a theta axis at the end of the Z plane to rotate the end-of-arm tooling. This makes SCARAs good for vertical assembly operations, such as inserting pins in holes without binding. However, the arm is essentially a lever, and that limits SCARAs’ reach: The joints are load points that need robust bearings and high-torque motors to handle the loads when the arm extends.
This Cartesian robot consists of basic system building blocks that were customized later. With such designs, OEMs can design controls and mechanical components separately and then tie them together with custom code for a timer to trigger a pick-and-place motion.
Six-axis robots move forward and back, up and down, and can yaw, pitch, and roll to offer more directional control than SCARAs. This is suitable for complex movements that simulate a human arm — reaching under something to grab a part and place it on a conveyor, for example. The additional range of movement also lets six-axis robots service a larger volume than SCARAs can. Six-axis robots often execute welding, palletizing, and machine tending. Programming their movements in 3D is complex, so software typically maps the motion to a set of world coordinates in which the origin sits on the pedestal’s first joint axis.
Picking between robot types
To pick a robot, first evaluate the application’s needs. That starts with profiling the job’s load, orientation, speed, travel, precision, environment and duty cycle, sometimes called LOSTPED parameters.
1. Load. A robot’s load capacity (defined by the manufacturer) must exceed the total weight of the payload, including any tooling, at the end of the robot arm. What limits SCARA and six-axis robots is that they support loads on extended arms. Consider a machining center that makes bearing assemblies of 100 kg or more. That payload exceeds the capabilities of all but the largest SCARA or six-axis robots. In contrast, a typical Cartesian robot can pick and place such loads with ease, because its support frame and bearings consistently support the entire range of motion.
This Cartesian robot is just as reliable as SCARAs and articulating robots, which have set parameters and directions of movement. In contrast, Cartesian robots are reconfigurable so manufacturers donâ™t have to buy new equipment when designs change.
Even when a heavy load is within a robot’s capacity, it can degrade accuracy. For example, picking and placing 50-kg items is within the payload range of both SCARA and Cartesian robots. But 50 kg is at the upper end of a typical SCARA’s capabilities, so it will take more costly controls and components to handle the torque. What’s more, typical SCARA robots can place heavy payloads to within 0.1 mm, as the weight deflects the arm and degrades the robot’s ability to consistently position the load with accuracy. But Cartesian robots with ball-screw actuators and well-spaced support bearings can repeatedly place 50 kg and heavier loads to within 10 µm.
2. Orientation depends on how the robot is mounted and how it situates parts or products being moved. The goal is to match the robot’s footprint to the work area. If a SCARA or six-axis robot’s floor or line-mounted pedestal creates an obstruction, then such robots may not be the best option. If the application only needs movement in a few axes, then small-frame Cartesian robots can mount overhead and out of the way. But for intricate part handling or work needing four or more axes of motion, a Cartesian robot’s framework can pose too many obstructions, and a small SCARA robot, sometimes requiring just 200 mm2 of space and four bolts on a pedestal, may be more suitable.
This Cartesian robot has controls that let operators safely enter the machine cage to teach it coordinates for picking and placing (sometimes just by pulling the end effector from point to point). That reduces training time for operators and reduces the need for engineers to alter machines already running.
Another factor is part orientation. SCARA and six-axis robots can rotate parts, an advantage for handling parts or tools at various angles and positions. To get similar flexibility, some Cartesian robots have subcomponents called feed modules that move light payloads in the Z axis. Typically, feed modules use a ball-screw thrust rod to move parts or tools along the Z axis in handling, pick-and-place, and feed applications. Cartesian robots can also incorporate rotary actuators to provide additional orientating capabilities.
3. Speed and travel. Along with load ratings, robot-manufacturer catalogs also list speed ratings. One key consideration when choosing robots for pick-and-place applications is acceleration times over significant distances. Cartesian robots can accelerate at 5 m/sec or more, rivaling the performance of SCARA and six-axis robots.
Cartesian robots also make sense when applications involve long spans. That’s because designers can quickly modify and extend Cartesian robots as needed with modules to 20-m long. Speed and distance are further customizable by choice of belt, linear motor, or ball-screw actuator. In contrast, articulating arms are typically predesigned for a given reach, such as 500 mm, for example.
End users can change a Cartesian robotâ™s mounting brackets, actuators, motors, and controls to meet changing application needs. For example, to accommodate new production requirements, an overhead-mounted Cartesian robot can change to a side mount.
SCARA and six-axis robots have predefined accuracy ratings that make it easy to determine their repeatability of movement. But these robots lock designers into one level of accuracy at the time of purchase. End users can upgrade Cartesian or gantry robots to myriad levels of accuracy by changing the actuator, even to 10 µm, with a ball screw. For less accuracy and to cut cost, end users can swap in a pneumatic or belt drive and a different actuator for 0.1-mm accuracy.
Precision is key in high-end applications such as machine tooling. Those Cartesian robots need better mechanical components such as precision-machined ball-rail tables and ball-screw actuators. For applications where SCARA and six-axis robot arms can’t maintain accuracy due to arm deflection, consider a Cartesian robot with high-precision linear bearings. The bearing spacing minimizes deflection so the end effector can be positioned more accurately.
Although small work envelopes favor SCARA or six-axis robots, sometimes these robots’ complexity and higher cost are unnecessary. One example where Cartesian robots work better is in a high-volume medical-pipette manufacturing application. Here, a robot takes pipettes from a mold and inserts them into a rack transported by a secondary automation machine. SCARA and six-axis robots are viable because 0.1-mm accuracy is sufficient in this application. But deflection is problematic when the robot handles smaller 3-mm pipettes. Plus, lack of room for a pedestal inside the cell favor gantry robots.
5. Environment. Two factors that dictate the best robot are the working envelope’s ambient environment and hazards in the space itself. A third consideration, whether a robot will go in a clean room, is generally not an issue because all robot types are manufactured in clean-room versions.
The pedestals of SCARA and six-axis robots tend to be compact, which is handy with limited floor space. But this may be irrelevant if installers can mount the robot’s support frame overhead or on a wall. In contrast, for applications with mechanical interference, as when a robot must reach into boxes to pull out parts, six-axis arms are usually most suitable. Six-axis robots typically cost more than Cartesians, but the expense is justified if there’s no way to execute the application without complex motion sequences.
On this robot, standardized components and diagnostics make maintenance less of a hassle. Cartesian robots are configurable so operators can also make basic changes in the field.
Environmental factors such as dust and dirt also affect robot selection. Bellows can cover SCARA and six-axis robot joints, and different types of seals protect Z-axis actuators. For clean rooms using air purges, Cartesian robots let designers enclose the linear actuators in an IP65 structure that minimizes entry of water and dust. Plus, high-performance seals can enclose many of the axes’ structural components.
6. Duty cycle. This is the amount of time it takes to complete one cycle of operation. Robots that run continuously 24/7 (as in high-throughput screening and pharmaceutical manufacturing) reach end of life sooner than those running only 8-hr days, five days a week. Clarify these issues in advance, and get robots with long lubrication intervals and low maintenance requirements to prevent aggravation later.
Robot programmability and controls
The most-suitable robot for an application also depends on the requirements for controls and programmability. All robot controls interpolate point-to-point, linear, or circular movements through path following and programmed speed, acceleration, and deceleration parameters. The options are entry-level and high-performance controls.
Engineers can design multiaxis Cartesian robots (as well as single-axis setups) with online selection and CAD tools that simplify sizing and specification.
Entry-level controls are generally available only with Cartesian robots. These operate with three degrees of freedom, which require less computer processing and programming sophistication than robots with more axes and degrees of freedom. Here, controls typically convert linear machine-movement sequences into X, Y, and Z Cartesian coordinates. However, a range of control options gives designers a choice between linear or circular interpolation. So if an application only needs point-to-point motion — to pick up paper cups, for example — then lower-level controls suffice.
High-performance controls process more degrees of freedom and convert machine-joint coordinates to the world coordinates necessary for complex rotary or circular movements. SCARA and six-axis robots usually have expensive, proprietary controls capable of circular interpolation and movement sequences — a justifiable expense when applications need complex profiling and path tracking.
Preparameterized control packages are another option. These come with about 80% of the programming already done. Predefined function blocks coordinate the motion of several axes. A software wizard tailors the program to specific applications and key parameters of the robot actuators, such ball-screw pitches. Simplified robotic mnemonic code lets the end user load and program the logic to save time.
With this software, end users aren’t locked into proprietary programs if they use a PLC that conforms to IEC 61131-3, which unifies the syntax and semantics of PLC languages. This means end users can use PLCs from different manufacturers and take their pick of programming with ladder diagrams, function-block diagrams, structured text, instruction list, or sequential function charts. Or programmers familiar with object-oriented languages can program PLCs without ladder logic or a proprietary language.
Feed modules like the Rexroth VKK provide SCARA-like control for moving lighter payloads on a Cartesian robotâ™s Z axis.
New capabilities in Cartesian-robot controls bridge this gap and let designers program in high-level programming languages such as C++ and Java. Then operators can make basic machine changes to Cartesian robots. Function blocks can be preloaded into drives, so even lower-level workers unfamiliar with programming can handle programming for simple pick-and-place applications, for example. Today’s Cartesian robots can also communicate with vision systems, conveyor trackers, and other sensors via protocol standards including Sercos, Ethernet/IP, and EtherCAT, which reduces the cost of controls.
SCARA and six-axis-robot features
Technological developments have advanced all robot types in recent years, but SCARA and six-axis robots:
• May come equipped to meet defined speed and motion specifications — and deliver higher performance right out of the box.
• May cost more because they come with expensive features such as proprietary controllers.
• Execute complicated tasks and require more programming for complex motion patterns.
• Take up less space and weigh less to fit a predefined footprint while delivering a specified speed.
• Exhibit less rigid arm extensions, because they are built to be as light as possible to carry heavier payloads.
• Come prebuilt for specific performance ratings (compared to Cartesian robots that designers can scale or tailor to specific needs).
Cartesian-robot features
Cartesian robots’ total cost of ownership has decreased 25% over the last five years due to pre-parameterized control packages, online design tools, and economies of scale. So now they are viable options for small to midsize manufacturers looking to automate storage-and-retrieval, pick-and-place, liquid-dispensing, packaging, and auxiliary machine-tool operations.
In addition, Cartesian robots:
• Consist of standardized components that combine into robots that cost less than purpose-built robots. This lets end users quickly assemble robots piece by piece rather than customize machines for every application. In some cases, standardized mounting brackets with centering rings replace traditional dowel pins on module guideways to further simplify assembly.
• Can be specified with online tools. Online software takes some of the confusion out of how to leverage the mix-and-match modularity of Cartesian robots. It lets engineers plan linear motion for single or multiaxis automation by entering the mass to be moved and the required stroke. In the past, designers ordered Cartesian-robot subcomponents using individual part numbers from different vendors. Now, engineers can often order integrated robot modules — including rails, servodrives, mechanical elements, and controls — with one vendor part number.
• Are safer. On legacy Cartesian robots, safety circuits connect to controls that introduce delays as they command the motor’s drive amplifier. Newer robots have intelligent servodrives instead, with safety circuits that accelerate response. These circuits also let Cartesian robots operate in reduced-torque mode, similar to the teach mode of SCARAs and six-axis robots. These modes let operators enter the robot’s safety cage and manually “teach” the robot coordinates to get a job done. To prevent injuries, robots in this mode shut down if it contacts the trainer.